1. Introduction
One simple and effective solution to enable the co-existence of 5G services and Fixed Satellite Service (FSS) receiving earth stations (ES) within the same geographical area is to retrofit the FSS earth station with a microwave bandpass filter (BPF) at the receiving frontend. Choosing the right BPF can help suppress the 5G interfering signals with the least impact on in-band FSS traffic, so that 5G network can be deployed with minimal restrictions.
There are multiple parameters to consider in designing the BPFs for each country. The fundamental issue is that the theory of 5G filter design must follow the law of physics which would not change from one design house to another. The key lies in the design parameters trade-offs. The BPFs provided by AsiaSat are specially designed by our technical team working closely with the experts from both the manufacturers and the broadcasters. The parameters considered by AsiaSat team include passband insertion loss (IL) which can translate to better link margins throughout the passband, along with other physical properties of the filter that allow for easy integration and better performance stability even under harsh environmental conditions.
In this article, we will first review some of the BPF design parameters that may help our customers select the right 5G-rejection BPF. The key specifications of a few leading BPFs in the market are set out in a table for easy comparison. We will also estimate and show the worst-case separation distances between a 5G base station and an FSS earth station. Through a comparison with other BPF models in the market, AsiaSat’s BPFs are demonstrated to be among the best performing BPFs in terms of the filter electrical and physical specifications.
2. The Considerations for Selecting a 5G-rejection BPF
In the Asia-Pacific region, C-band FSS has played a much-needed role in providing connectivity to underserved communities and improving people’s social and economic lives. The major applications have included direct-to-home (DTH) videos, two-way VSAT communications, satellite news gathering, TV content contributions and distributions, etc. Various kind of C-band satellite dishes with differently-sized apertures can be found across the region. However, the recently deployed 5G networks are encroaching upon a portion of C-band spectrum and are poised to take away more spectrum from satellite services. Interested readers can look up the detailed 5G to FSS interference types and symptoms in [1].
To limit the impact from 5G interference, one effective and economical way is to install a 5G-rejection BPF between the low-noise block down-converter (LNB) input and the satellite antenna output. It is important to choose the right 5G rejection BPF to fit your needs. Intuitively, the higher rejection level of a BPF will significantly reduce interference level to the FSS traffic and allow a closer co-existing distance with the neighbouring 5G base stations. However, the BPF rejection parameter is a design trade-off with the other design specifications of the BPF, such as the passband IL (which lead to the link margin and the antenna size’s change), the filter physical length and the mass.
The microwave BPF passband IL is mainly affected by the microwave resonator technology used, filter bandwidth, filter order (the number of system poles or resonator cavities used), and the manufacturing techniques. The commonly used resonator technology for a C-band satellite receiving preselection BPF is coaxial re-entrant cavity. The performance of the resonator cavities is evaluated by their unloaded-Q factor (Qu), which can vary from a few hundred up to several thousand, depending on the cavity size, the silver-plating quality and the tuning element status, etc. With the same metal conductivity, a bigger resonator cavity usually provides a higher Qu and hence a lowered IL from the filter realized by such resonators. However, using bigger resonator cavities also means the filter will be bulkier and heavier that may lead to structural support issues for the installed system of the ES.
With the other parameters fixed, the higher filter order of a BPF will result in the higher out-of-band rejection as well as the passband IL. We can illustrate this phenomenon in Figure 1, where the rejection and IL performances for the all-pole BPFs of filter order = 6, 8 and 12 are shown. When the filter topologies and resonator Qu are fixed (Qu = 2000), we will find the 12-pole BPF provides the sharpest out-of-band rejection roll-off (Figure 1(b)) but also the worst passband IL (Figure 1(a)). Therefore, a higher rejection level on the 5G band can be achieved at the cost of a degraded IL on the FSS passband, and even the extra size and mass of the filter, since more and bigger resonator cavities may have to be used.
The illustration in Figure 1 can be viewed from another angle. If the required minimum rejection level, e.g. 40 dB, is known to sufficiently suppress a known 5G-interference source nearby, we can find that at least 65 MHz, 135 MHz and 240 MHz guard band separations (measured from the passband’s lower edge at 3.7 GHz) would be needed for the 12, 8 and 6-pole BPFs shown in Figure 1(b) respectively. That is, if there is sufficient guard band, we can choose a BPF that meets the rejection specification with the lowest IL penalty and a more compact design (with fewer and smaller resonator cavities) that suits the existing ES hardware configuration.
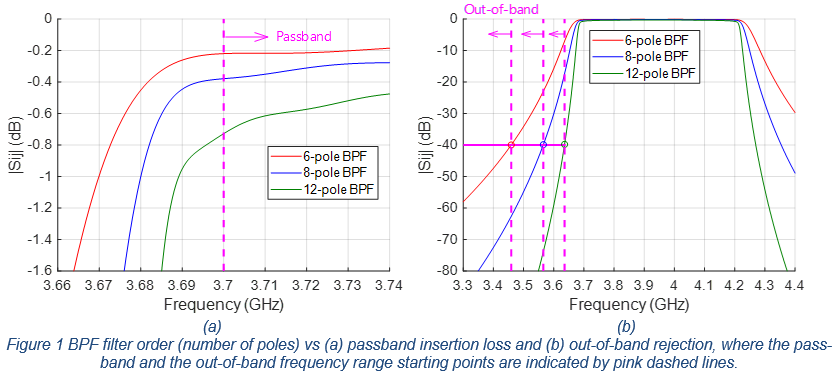
The extra insertion loss imposed by the BPF can be translated into an equivalent system G/T degradation of the receiving terminal. For example, if the BPF IL is 1.0 dB, along with the existing lossy components (i.e. the antenna, OMT, feeder waveguide and LNB on the RF signal path), it can cause more than 2.5 dB system G/T degradation. The worsened G/T can be further translated into a lowered carrier-to-noise (C/N) ratio, and hence a lowered Service-Level Agreement (SLA) of the satellite link. For example, if the aforementioned satellite link C/N ratio is lowered by 2 dB after the BPF installation, the link MODCOD used may have to be lowered from 8PSK 3/4 to QPSK 5/6 to maintain the same link margin. Meanwhile, we may have to decrease the data rate from 70 Mbps to 50 Mbps to match the link C/N ratio; or we can compensate the G/T degradation and maintain the data rate by increasing the antenna size. Either way, the result is additional cost to the customer.
On the other hand, we must also take the filter physical length and mass into account before the ES antenna modification. Many C-band antennas used in the region are the front-fed type as shown in Figure 2 (a), where the feed horn and the LNBs are directly mounted on top of a simple tripod, facing the main reflector. If the installed 5G-rejection BPF is too heavy, it can overload the tripod struts and even defocus the parabolic antenna, causing further G/T degradations. If the filter profile is too big, it may significantly increase the wind resistance of the antenna and impose a higher risk of damage during typhoon season which affects many Asia-Pacific countries.
The backside-fed C-band antenna is less sensitive to the filter mass, since the BPF is to be installed on the backside of the main reflector, typically inside a hub assembly. However, many such antenna hubs are designed to have very limited room as shown in Figure 2 (b). If the BPF is too long, an extra 90° waveguide bend must be used to accommodate the BPF on the 90° polarisation path, and further, the LNB attached to it may have to be left outside of the hub room and exposed to elements.
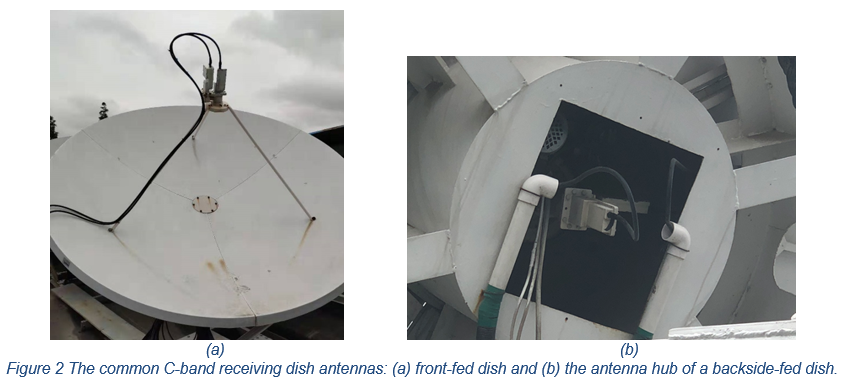
If the BPF is left exposed to open air like the one in Figure 2 (a), we must also consider the degree of protection offered by the filter body. Since many Asia-Pacific countries are marked by heavy rains and high humidity with salty air corrosion, we suggest using BPFs that adopt higher IP6X standard.
To summarise, when selecting a proper 5G-rejection BPF, we must consider the passband IL, filter length and mass together with the out-of-band rejection level. The suggested BPF selection process would involve the steps as follows:
- First, the passband and rejection bands of the selected BPFs must match the respective local FSS and 5G bands. In the Asia-Pacific region, the C-band 5G spectrum may end at different frequencies, e.g. 3.6 GHz, 3.65 GHz, 3.7 GHz or 3.8 GHz, for different countries. AsiaSat has developed BPF-3700S, BPF-3646, BPF-3700T, BPF-3800S, BPF-3800R, BPF-3900S and BPF-4230 series to best accommodate the various 5G-FSS co-existence environments [2]. It is also worth mentioning that, if the satellite ES is located close to a busy air route, the capability to reject the airplane altimeter radar spectrum (4.2-4.4 GHz) may be better to be integrated on the same BPF, like the BPF-3800R or BPF-4230 model.
- The second step is the trade-off between out-of-band rejection and passband IL. If the 5G interference is severe and the survivability is the first priority, then the rejection level specification must be weighted the highest. If needed, even the whole antenna dish may be replaced to accommodate the BPF. However, for most FSS earth stations, the existing antenna must be kept and the resultant satellite link degradation must be affordable. A BPF with the balanced rejection-IL performance is thus the desired selection. To reach this goal, the 5G interference level must be estimated or measured at the ES. The margin must be reserved for the future 5G deployment as well as the coordination conditions in the neighbourhood.
- The third step is the trade-off between the electrical performance with the critical physical specifications of the BPF, such as the filter length and mass. After all, the purchased filter must be able to be fitted on the existing antenna hardware without causing extra problems such as defocusing the antenna or endangering the mechanical structures and lowering the link availability in bad weathers. The above two steps may have to be iterated several rounds to reach the optimal BPF selection.
Comparison of AsiaSat BPFs with Other 5G-Rejection Filters
In this section, we compare BPF-3800S and BPF-3700T, two BPF models provided by AsiaSat [2] together with other BPF models in the market, which we shall call A, B, C, D and E, whose specifications can be found online. As discussed in the last section, the key specifications compared are the passband frequency range, passband IL, out-of-band rejection, filter length, mass and the filter body protection level. Since not all BPFs compared share the same passband frequency range, the out-of-band rejection levels are thus compared at the frequencies relative to the lower band edge (LBE) of the BPF passband.
The specifications of the compared BPFs are shown in Table 1, where the rejections along with other listed specifications are taken from their respective BPF datasheets without interpolation or other manipulations. The rejection against guard band separation data can be plotted on a separate chart as shown in Figure 3 (a).
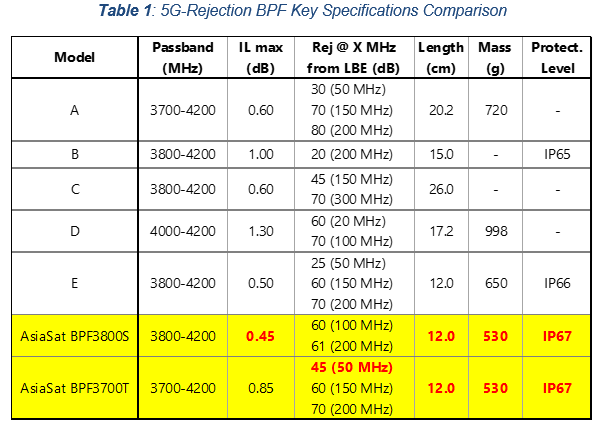
It can be seen that when it comes to 5G-rejection BPF, there is no one-size-fits-all. Different countries can have different requirements on the frequency allocation for the FSS and 5G networks. If the FSS-5G guard band separation is 20 MHz as in the U.S., BPF model D has to be used at the cost of much bulkier profile and significant IL. However, if the FSS earth station is located in South Korea or Australia with 100 MHz FSS-5G separation, AsiaSat BPF-3800S can be used to provide more than 60 dB rejection with the lowest passband IL, smallest filter length / mass as well as the highest degree of filter body protection among all BPFs compared. If the guard band is 50 MHz as in Singapore, AsiaSat-3700T can be selected to balance the 5G rejection level with the in-band IL performance and other specifications.
We also highlight the area with guard band separation < 100 MHz in Figure 3 (a), because in most countries around the world, the FSS-5G guard band separations are equal or less than 100 MHz. Thus, even higher rejection levels achieved beyond the 100 MHz range will contribute negligibly to the overall 5G interference rejection.
The required FSS-5G separation distance (in meter) can be estimated and plotted on a chart against the guard band separations (in MHz), if the rejection level of the BPF used is known. However, this type of chart can be confusing and misleading if the assumptions are not clearly laid out. In Figure 3 (b) we have made such a comparison with the worst case FSS-5G co-existence scenario. It assumes that the 5G base station EIRP is 78 dBm (200W AAU RF output power plus 25 dB 5G antenna gain); the angular separation (illustrated in Figure 4 (b)) between satellite ES and 5G site is 10° with 7 dB off-axis receiving antenna gain from the ES; the satellite LNB maximum allowed input power is -60 dBm; and only the LNB saturation interference [1] is considered.
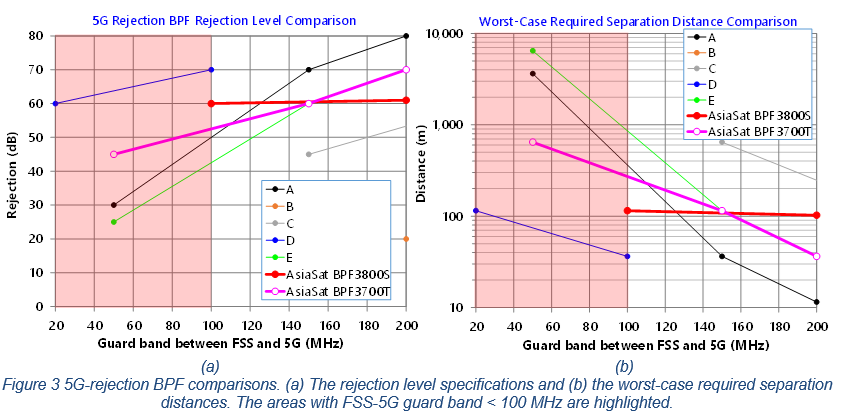
Figure 3 (b) shows that AsiaSat BPF-3800S and BPF-3700T are among the best-performing BPFs with the shortest separation distances to co-exist with a 5G base station. In a real-world co-existing scenario, a satellite ES may rarely point directly to a 5G base station (within 10° vicinity of the ES antenna boresight), and a 5G base station may also have little chance to beam all its Tx power to a neighbouring satellite ES. Therefore, the actual required co-existence separation distances can be shorter than those shown in Figure 3 (b). The interested readers can refer to [3] for a real-world BPF installation troubleshoot case.
If the BPF 5G-band rejection level is known, we can also estimate the required separation distance (in meter) against the angular separation (in deg) between the satellite ES antenna boresight and a 5G base station as shown in Figure 4 (a). It can be seen when the angular separation increases, the required co-existence separation distance can be decreased. In most real-world cases, the angular separation between an FSS ES and a 5G base station is large.
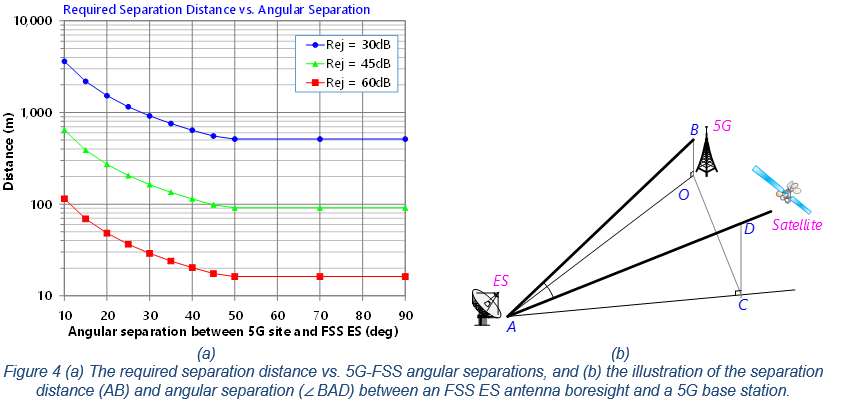
4. Conclusion
At a recent webinar [4], one of the speakers looked at the co-existence challenges and reviewed the BPFs that are currently available in the market, including the ones provided by AsiaSat. We note that the “Separation Distance Versus Guard-Band” chart in [4] that was used to determine the separation distance for comparing different BPFs was misleading and could lead to incorrect buying decisions. That is why we have replotted the required separation distance curves setting out clearly the underlying assumptions in this article. We also reviewed a few specifications related to the selection and the use of a BPF and examined at the trade-offs between filter rejection, insertion loss and other key electrical and physical performance.
As you can see from the charts shown above, AsiaSat BPFs can accommodate the different frequency bands used for 5G services in all major markets in the Asia-Pacific region. Our comprehensive range of specially design and optimized BPFs stand out from among competing models in the market. Compared with other BPFs, AsiaSat BPFs have the most compact profile, highest filter body protection standard and balanced electrical performance in both out-of-band and in-band frequencies.
References
[1]. “AsiaSat’s 5G Interference Rejection Bandpass Filter,” AsiaSat White Paper, Dec. 2019. Available online at https://www.asiasat.com/sites/default/files/AsiaSat_5G_rejection_BPF_whitepaper_Dec_2019.pdf.
[2]. AsiaSat 5G Interference Rejection Bandpass Filter Homepage: https://www.asiasat.com/innovations/5g/5Gfilter.
[3]. “AsiaSat: A real-world case in solving a problem with BPF installation,” AsiaSat White Paper, Apr. 2020. Available online at https://www.asiasat.com/sites/default/files/AsiaSat%20White%20Paper_A%20Real-World%20Case%20in%20Solving%20a%20Problem%20with%20BPF%20Installation.pdf.
[4]. “The economic realities of C-band in Asia-Pacific and globally,” APSCC 2020 webinar, Oct. 2020. Available online at https://apsccsat.com/wp-content/uploads/2019/09/APSCC-2020_Intelsat-Juwad-Mohaned-PPT.pdf.